By Maxence Cordiez, energy engineer
The subject of hydrogen regularly comes up in debates on the energy transition, whether to reduce dependence on oil in the 70s or greenhouse gas emissions in the 90s. However, low-carbon hydrogen has hardly developed to date, due to a number of constraints and limitations, which should not be underestimated, surrounding its production, storage, transport and use.
Things have been changing in recent years, however, with a number of countries such as France[1], Germany[2], Spain[3], the European Union[4], Japan[5], Australia[6]… having almost simultaneously published their deployment strategies for this sector.
The objectives and approach to this issue vary significantly from country to country, with some countries seeing it as a way to decarbonize certain uses that are difficult to electrify, and to compensate for the intermittent nature of wind and solar production by relying in part on hydrogen imports (Germany), while others are considering meeting their own needs to decarbonize sectors that are difficult to electrify (France).
In this article, we’ll look at the stakes involved in the emergence of a hydrogen industry, as well as some of its limitations.
What is hydrogen?
Hydrogen – or should we say dihydrogen – is a reducing molecule (i.e. able to give up electrons, unlike an oxidizing agent) and a fuel. French industry currently consumes just under a million tonnes a year, in the following sectors: oil refining (60%), fertilizer production (25%), chemicals (10%), miscellaneous applications (4%) and metallurgy (1%)[7].
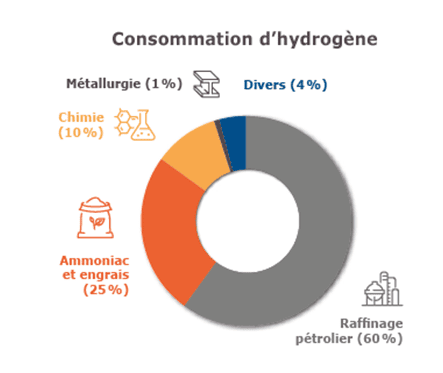
While applications in oil refining are set to decline over time in line with the goal of carbon neutrality and the depletion of fossil fuels, the others are set to continue, and new applications (in industry and mobility) are expected to emerge. Achieving the goal of carbon neutrality should therefore boost demand for low-carbon hydrogen.
France’s 2020 hydrogen strategy calls for the installation of 6.5 GW of electrolyzers by 2030, which would enable the production of nearly one million tonnes ofH2 (provided the electrolyzers can operate with a high load factor, otherwise less).
Today, 95% of the hydrogen consumed in France is of fossil origin, and is produced in four ways. For the first three, hydrogen is a co-product:
- 40% by hydrocarbon oxidation (by-product of the oil industry),
- 15% by coal gasification (co-product of coke production),
- 5% by electrolysis of brine (by-product of chlorine production),
- 40% by steam reforming of methane.
It is only via the last route, methane steam reforming, that hydrogen is produced for its own sake, and could therefore compete directly with hydrogen produced by electrolysis.
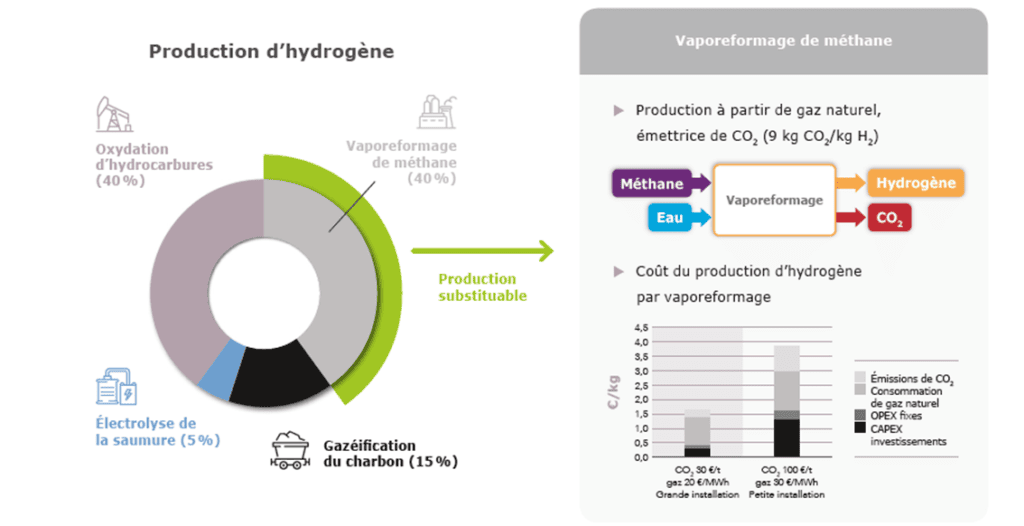
Regardless of hydrogen supply and demand (low-carbon or otherwise), as long as the steel industry continues to consume coke to reduce ore to metal, hydrogen will be co-produced with the coke (with significant CO2 emissions). To reduce the production of carbonated hydrogen, we need to reduce the production of coke, and therefore decarbonize the steel industry… for example, by substituting coke with hydrogen (low-carbon).
Hydrogen production in France emits around 10 MtCO2/year, corresponding to 2-3% of national emissions.
Electrolysis will generate substantial electricity needs
In France, in the short term, 400,000 tonnes of hydrogen (the volume currently produced by vapor-reforming methane) can be decarbonized by electrolysis of water using low-carbon electricity. In the medium term, new uses for low-carbon hydrogen could emerge, production permitting.
Without going into the economic aspects just yet, one of the limits of hydrogen production lies in the production of electricity. Indeed, without taking into account energy losses during the hydrogen compression, transport and storage stages, the efficiency of the electricity-to-hydrogen conversion process expected by 2030 is 63-71% (values in lower calorific value, LCV)[8]. For example, a 900 MW nuclear reactor operating at a load factor of 80% would produce around 128 kilotonnes of hydrogen (ktH2) per year. A 3 MW onshore wind turbine with a load factor of 25%[9] will produce 133 tH2/year. As a result, just over 3 x 900 MW reactors or 3,000 x 3 MW onshore wind turbines would be needed to decarbonize the 400,000 tonnes of hydrogen currently produced by methane steam reforming.[10].
Hydrogen should be reserved for sectors that cannot be decarbonized otherwise.
Let’s assume that hydrogen engines for airliners exist, are mature and can handle all routes up to long-haul (this is not the caseIn fact, the aeronautics industry has no plans at this stage to fly long-haul aircraft on hydrogen, as this energy carrier is being considered for short- and medium-haul aircraft.). Replacing the 6.8 Mt of jet fuel consumed in France in 2018[11] would require 2.5 MtH2, the production of which would mobilize on the order of 11 EPR nuclear reactors or 19,000 3 MW wind turbines (taking only hydrogen production into account, i.e. without the compression, transport and storage stages).
Another example: heavy goods vehicles consumed 6 Mt of diesel in France in 2018.[12]. Decarbonizing this traffic on a like-for-like basis (without reducing demand) with hydrogen alone would require around 9 EPRs or 16,000 wind turbines. Not to mention passenger cars, which consumed 16 Mt of diesel.[13] and 6.6 Mt of gasoline[14] in 2018…
These order-of-magnitude calculations show the massive electricity requirements involved in decarbonizing the hydrogen currently consumed in France, as well as its extension to new uses.. As is often the case when it comes to energy, this observation must not be used as a pretext for Manichean positions. Whether it’s to say that hydrogen has no future because of its electrical needs (we already consume it, and we’ll need it to decarbonize new uses), or that all we need to do is build dozens of EPRs or tens of thousands of wind turbines dedicated to hydrogen production (which isn’t realistic, especially considering that we’re already going to have to step up investment to renew the current power generation fleet).
Hydrogen will be necessary to reduce our oil consumption in certain sectors, but the electrical needs it generates mean that :
- reserve it for uses that it alone can decarbonize: industry and heavy mobility (i.e. cars should be excluded from its scope, as they can be electrified by batteries),
- reduce these uses (in particular, reduce air traffic),
- rapidly increase the number of low-carbon power plants.
In short, we need to be sober and concentrate the use of hydrogen on essential applications that would not otherwise be decarbonizable.
Rejoignez les 40000 abonné(e)s à notre newsletter
Chaque semaine, nous filtrons le superflu pour vous offrir l’essentiel, fiable et sourcé
Hydrogen will remain an expensive energy carrier
Hydrogen produced by electrolysis will necessarily be more expensive than hydrogen produced from fossil fuels, and than the direct use of fossil fuels at their current cost. This additional cost is permanent because it has a physical origin. Any change of energy vector (heat -> electricity; electricity -> hydrogen; methane -> hydrogen…) leads to energy losses and requires infrastructure. For example, to convert gas into electricity, you need a gas-fired power plant (an infrastructure that isn’t free), with an efficiency of around sixty percent (i.e. 30-40% losses).
Since hydrogen production by electrolysis requires two changes of energy carrier – with electricity as an intermediate carrier – it will necessarily be more expensive than hydrogen from methane steam reforming, and a fortiori than fossil fuels used directly.
Note that imposing a sufficiently high price on CO2 emissions and/or increasing the price of fossil fuels may eventually make electrolysis production more profitable. However, in this hypothesis, the reversal of competitiveness in favor of electrolytic hydrogen would be achieved by increasing the cost of fossil fuels. This doesn’t change the fact that the cost of access to energy would increase compared to today.
Because of production losses, the synthesis of hydrogen by electrolysis and the promotion of its use only make sense from a climate point of view in countries where the electricity mix is already low-carbon. If this is not the case, it makes more economic and ecological sense to decarbonize power generation first. This means that while most European countries (including Germany) will be entering the hydrogen market in order to keep pace with the others, this positioning only makes real sense for the climate in a limited number of countries (France, Norway, Sweden, Switzerland…).
Reducing the cost of electrolytic hydrogen
If electrolytic hydrogen is to play a significant role in achieving the goal of carbon neutrality, it will also be clear from the above that it is essential to minimize its competitive disadvantage in relation to fossil fuels. Two levers make this possible.
The first way to reduce the cost of low-carbon hydrogen is to use electrolyzers with high charge factors.[15]. The higher the hydrogen production per electrolyzer, the more the fixed costs will be spread over a large production run. This first observation is incompatible with the idea of producing hydrogen solely from marginal surpluses of wind and solar power, unless we have overcapacity, part of whose production would not be recoverable and would have to be capped. Indeed, the constraints on the load factor of electrolyzers will limit the installed electrolysis capacity. In other words, we’re not going to install an electrolyser that will only run for a few hours a year to capture production peaks. Certain surpluses of electricity production will remain unprofitable in any case, and they will be all the higher the more electricity production is uncontrollable and synchronized (i.e. everything is produced more or less at the same time).
The second lever is to reduce the cost of electricity production. This cost should not be confused – as is often the case – with the market price of electricity (which can be low, zero or even negative). Generating electricity is never free. The difference between the cost of production and the market value of electricity is compensated by the taxpayer, via remuneration supplements (for renewable energies) and a capacity market (for controllable energies). So, minimizing market costs is not enough: it’s the overall cost of access to energy for the consumer-taxpayer that needs to be minimized.
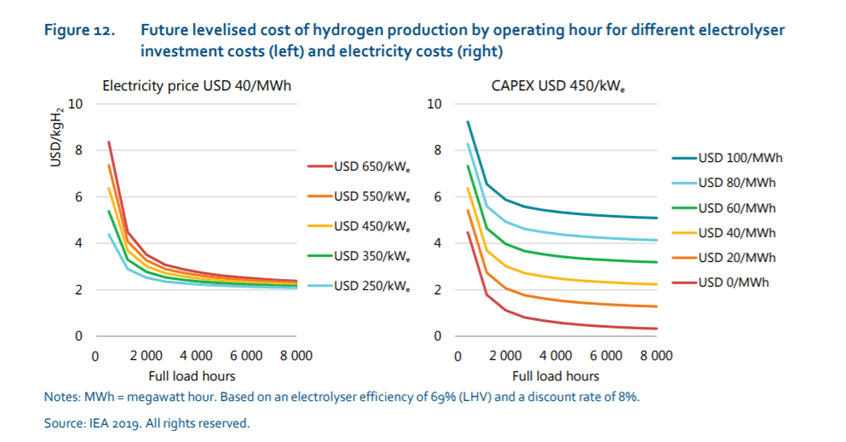
For these reasons, the future of the hydrogen industry and its contribution to the decarbonization of the industrial and heavy mobility sectors depends, in particular, on the acceptance of nuclear energy as one of the means of powering electrolyzers in countries without significant hydroelectric and offshore wind power capacity (whose load factor is higher and cost lower than onshore wind power). Otherwise, low load factors will make hydrogen expensive, limiting its potential.
Finally, beyond the question of cost alone, the scale of future hydrogen requirements means that we need to be able to maximize production, particularly in relation to the number of electrolyzers installed. Here again, this aspect encourages us to seek to operate them with a high load factor.
Conclusion
The question of hydrogen and its derivatives is a good illustration of the need for a non-Manichean approach to energy issues. Hydrogen is not a miraculous solution that will enable us to decarbonize all our energy uses at a cost comparable to the current cost of energy. Yet it is an energy vector that will be needed to decarbonize certain activities that would otherwise be difficult to decarbonize.
This question also illustrates another parameter that is too often overlooked: the energy transition will not lead to a reduction in the cost of energy. It will not even maintain it at its current level. If this were the case, and it were economically viable to decarbonize our economies, we would already have done so. Achieving carbon neutrality will entail additional costs. Some will be temporary (the time it takes to acquire equipment, the time it takes to develop skills in certain areas and bring costs down), while others will be permanent, due to energy systems that are generally less efficient than those based on fossil fuels that are easily accessible, energetically dense and naturally storable.These observations go far beyond the subject of hydrogen alone. To maximize our chances of achieving carbon neutrality, we need to be aware of these difficulties and not compound them with sub-optimal decarbonization strategies.
When it comes to hydrogen, there are three major mistakes to avoid. The first would be to overestimate hydrogen’s potential (in terms of available volume, competitiveness and speed of deployment). The second would be to limit production to renewable energies alone, which would lead to high costs and limit electrolyser production. Finally, the third would be to confuse the objective of decarbonizing industry and heavy mobility (which would mean minimizing the competitive disadvantage of electrolytic hydrogen compared with fossil hydrogen) with that of having an electricity mix based mainly on wind and solar power, in which hydrogen would be used as storage to compensate for their intermittency (which would lead to uncompetitive hydrogen).
As is so often the case when it comes to energy, technology, long-term strategy, economics and social issues are all intertwined in a complex mix. It is essential to be aware of them and to take them into account correctly, lucidly and without preconceptions, in order to optimize the path to carbon neutrality.
[1] French government, Stratégie nationale pour le développement de l’hydrogène décarboné en France, September 2020, https://www.ecologie.gouv.fr/sites/default/files/DP%20-%20Strat%C3%A9gie%20nationale%20pour%20le%20d%C3%A9veloppement%20de%20l%27hydrog%C3%A8ne%20d%C3%A9carbon%C3%A9%20en%20France.pdf
[2] German Federal Government, The National Hydrogen Strategy, June 2020, https://www.bmwi.de/Redaktion/EN/Publikationen/Energie/the-national-hydrogen-strategy.pdf?__blob=publicationFile&v=6
[3] Spanish Government, Hoja de Ruta del Hidrógeno: una apuesta por el hidrógeno renovable, October 20, https://www.miteco.gob.es/es/prensa/201006nphojaderutah2_tcm30-513813.pdf
[4] European Commission, A hydrogen strategy for a climate-neutral Europe, July 2020
[5] Government of Japan, Basic Hydrogen Strategy, December 2017
[6] Australian Government, Australia’s national hydrogen strategy, 2019
[7] RTE, The transition to low-carbon hydrogen, January 2020, page 14
[8] IEA, The Future of Hydrogen, 2019, page 45
[9] Calculated from RTE’s 2019 and 2020 electricity balances
[10] Note that the second case would require greater electrolysis power, which would operate with a lower load factor, due to the greater variability of wind power.
[11] INSEE, https://www.insee.fr/fr/statistiques/2119673
[12] Statista, https://fr.statista.com/statistiques/487045/consommation-gazole-poids-lourds-francais-france/
[13] Statista, https://fr.statista.com/statistiques/486956/gazole-essence-voitures-particulieres-france/
[14] Statista, https://fr.statista.com/statistiques/486845/consommation-essence-voitures-particulieres-france/
[15] IEA, The Future of Hydrogen, June 2019, page 47